Material Analysis with X-ray Scattering Tensor Tomography
- Aditya Verma
- Mar 20, 2024
- 8 min read
TLDR
The Challenge of Accurately Modeling Heterogeneous Materials
Limitations of Current Micro-scale Imaging Technologies in Comprehensive Material Analysis
Integrating X-ray Scattering Tensor Tomography with Finite Element Modeling
Advancing Material Property Predictions with High-Resolution Imaging and Numerical Modeling
The Challenge of Accurately Modeling Heterogeneous Materials

The accurate modeling of heterogeneous materials, such as carbon fiber-reinforced polymers (CFRPs), presents a significant challenge in the field of materials science and engineering. These materials are increasingly indispensable across various industries, including automotive, aerospace, and construction, owing to their exceptional mechanical properties. However, the complexity of their micro-scale structures, which are critical in determining their macroscopic properties like stiffness and strength, necessitates advanced imaging and modeling techniques for comprehensive analysis.
Traditionally, X-ray micro-computed tomography (micro-CT) has been the state-of-the-art method for imaging these complex materials. It allows for the visualization and analysis of micro-scale features within a material, providing valuable insights into its structural integrity and performance. Yet, this method is not without its limitations. The primary challenges include the struggle with low contrast in carbon or natural fiber-reinforced composites, which complicates fiber segmentation for further analysis. Additionally, there's a significant trade-off between the desired field-of-view (FOV) and the length scale of interest. Achieving a comprehensive view of the material often requires the stitching together of multiple scans, leading to massive datasets that are daunting in terms of both data processing and experiment duration.
The introduction of small-angle X-ray scattering (SAXS) imaging offers a promising alternative. Unlike traditional methods, SAXS does not require resolving individual fibers, thus easing spatial resolution requirements and enabling the analysis of the mechanical properties of fiber-reinforced composites in a comprehensive volumetric FOV. This method effectively captures micro-structural details, such as fiber orientations and distributions, that are orders of magnitude smaller than the voxel size, through the measurement and decoding of local SAXS signals.
Despite the advances in imaging technologies, the transfer of this detailed SAXS data into a numerical model capable of accurately predicting actual material properties remains a significant hurdle. This challenge is compounded when considering materials like CFRPs, where the fiber orientation plays a crucial role in their mechanical behavior. The traditional image-based modeling strategies, which often involve finite element models constructed from image datasets, are limited by the resolution of the imaging technique and the computational resources required to process large datasets.
The need for a new approach that can overcome these limitations is clear. Such a method would not only need to accurately capture the complex micro-architecture of heterogeneous materials but also efficiently translate this information into a predictive model for their mechanical properties. Addressing this challenge is crucial for advancing our understanding and utilization of these materials in various applications, pushing the boundaries of what's possible in materials design and engineering.
Limitations of Current Micro-scale Imaging Technologies in Comprehensive Material Analysis
In the realm of materials science and engineering, the detailed analysis of heterogeneous materials like carbon fiber-reinforced polymers (CFRPs) is pivotal. These materials' superior mechanical properties, crucial for applications across aerospace, automotive, and construction sectors, are inherently tied to their complex micro-scale structures. Yet, the task of accurately characterizing and modeling these materials faces significant hurdles due to limitations in current micro-scale imaging technologies.
X-ray micro-computed tomography (micro-CT) has emerged as a popular choice for imaging heterogeneous materials, providing high-resolution insights into their micro-structures. This technology's ability to non-destructively examine the internal structure of materials offers a clear advantage. However, it's not without its limitations, which significantly impact the comprehensive analysis and numerical modeling of materials:
Low Contrast in Fiber-reinforced Composites: Traditional absorption-based X-ray imaging methods struggle with low contrast in carbon or natural fiber-reinforced composites, complicating fiber segmentation for further analysis. This issue stems from the materials' inherent properties, where the difference in X-ray absorption between the fiber and matrix phases is minimal, making it challenging to distinguish between them clearly.
Trade-off Between FOV and Resolution: Achieving a detailed view of the material's micro-architecture often requires a compromise between the field-of-view (FOV) and the resolution. To capture the orientations of carbon fibers, for example, a large number of high-resolution micro-CT scans may be needed, resulting in massive datasets. This not only poses challenges in data processing but also significantly extends experiment durations.
Massive Data Sets: The need to stitch together multiple scans to achieve a comprehensive view of the material results in enormous datasets, often in the range of terabytes. Managing, processing, and analyzing such volumes of data demand considerable computational resources and time, posing a significant bottleneck in the analysis process.
Time-Consuming Acquisition and Analysis: The process of acquiring and reconstructing micro-CT scans, coupled with the subsequent fiber orientation analysis and finite element modeling, is inherently time-consuming. This extends the timeline for material characterization and delays the feedback loop into material design and testing processes.
These limitations highlight a critical gap in the current capabilities of micro-scale imaging technologies for the comprehensive analysis of heterogeneous materials. There's a pressing need for advanced imaging methods that can overcome these challenges, offering faster, more efficient, and more accurate characterization and modeling capabilities. The integration of small-angle X-ray scattering (SAXS) imaging represents a promising advancement in this direction, potentially addressing the shortcomings of conventional methods by allowing for comprehensive volumetric analysis without the need for high spatial resolution or extensive data stitching.
Integrating X-ray Scattering Tensor Tomography with Finite Element Modeling
The quest for a more refined and comprehensive analysis of heterogeneous materials, particularly carbon fiber-reinforced polymers (CFRPs), necessitates overcoming the substantial limitations posed by conventional imaging technologies. Enter the innovative approach of integrating X-ray Scattering Tensor Tomography (XSTT) with Finite Element Modeling (FEM), a leap forward in accurately predicting material properties by leveraging detailed micro-architectural data.
X-ray Scattering Tensor Tomography: A Paradigm Shift
The advent of X-ray scattering tensor tomography (XSTT) represents a significant advancement in imaging technology for materials science. Unlike traditional methods, XSTT transcends the limitations of spatial resolution and contrast issues by not requiring the resolution of individual fibers. This method leverages improved X-ray optical elements and acquisition methods, allowing for the complete analysis of micro-architecture samples in the dimension of centimeters within minutes, a stark contrast to the lengthy and data-intensive processes associated with micro-CT.
The Core of XSTT
At the heart of XSTT is the ability to capture and decode local small-angle X-ray scattering signals, providing a detailed map of fiber orientations and distributions far below the voxel size. This method is particularly adept at analyzing the mechanical properties of fiber-reinforced composites, offering a comprehensive volumetric field-of-view that traditional methods cannot achieve. The critical leap forward lies in the ability to transform this detailed scattering data into a numerical model that can predict actual material properties accurately.
Finite Element Modeling: Bridging the Gap
The integration of XSTT with finite element modeling (FEM) bridges the gap between micro-scale imaging and macroscopic property prediction. This approach allows for the numerical prediction of mechanical properties based on imaging data with a voxel size of approximately 100 μm. The innovation lies in constructing the material's constitutive law based on fiber orientation, X-ray scattering anisotropy, and intensity, as revealed by XSTT. By combining advances in X-ray imaging with the presented material model, this methodology opens the door for accurate tensile modulus prediction and beyond.
Overcoming Traditional Challenges
This integrated approach addresses the traditional challenges head-on. By eliminating the need for high-resolution imaging to resolve fibers, the method significantly reduces the data volume, processing time, and the overall complexity of material analysis. Moreover, it allows for the analysis of volumes of interest between three to six orders of magnitude larger than what conventional carbon fiber orientation image-based models can cover.
The Promise of Integrated XSTT and FEM
The promise of combining XSTT with FEM lies not just in overcoming existing limitations but in setting a new standard for material analysis. This methodology not only enhances our ability to study materials with intricate micro-structures but also significantly improves the efficiency and accuracy of modeling efforts. It marks a significant step toward more sophisticated and practical analyses of heterogeneous materials, ultimately facilitating the development of materials with optimized properties tailored for specific applications.
In essence, the integration of X-ray scattering tensor tomography with finite element modeling represents a transformative approach in materials science, offering a powerful tool for the detailed analysis and accurate prediction of material properties. This advancement underscores the importance of innovative imaging and modeling techniques in driving the field forward, enabling new discoveries and applications in material design and engineering.
Advancing Material Property Predictions with High-Resolution Imaging and Numerical Modeling
The integration of X-ray Scattering Tensor Tomography (XSTT) with Finite Element Modeling (FEM) heralds a significant breakthrough in the predictive modeling of heterogeneous materials, such as carbon fiber-reinforced polymers. This innovative approach, by leveraging the detailed micro-scale information obtained from XSTT, enables a more accurate prediction of material properties, overcoming the inherent limitations of conventional imaging techniques.
The Advantages of XSTT in Material Analysis
X-ray scattering tensor tomography offers unparalleled insights into the micro-architecture of materials. By providing detailed information on fiber orientations and distributions at a micro-scale, XSTT enables the construction of a more accurate numerical model of the material. This method allows for the analysis of samples in the dimension of centimeters within minutes, significantly enhancing the efficiency of material characterization.
Enhancing Predictive Modeling through FEM Integration
The integration of XSTT data into FEM represents a significant advancement in the field of material science. By utilizing the detailed scattering data, researchers can construct finite element models that accurately reflect the material's microstructural characteristics. This approach allows for the precise prediction of mechanical properties, such as tensile modulus, by accounting for the actual fiber orientation and distribution within the material.
Addressing Traditional Limitations
Traditional imaging methods, such as micro-CT, are limited by their inability to capture the complete micro-architecture of materials without extensive data stitching and processing. The integration of XSTT with FEM addresses these limitations by providing a comprehensive analysis of the material's micro-architecture without the need for high spatial resolution or extensive computational resources. This methodology enables the analysis of larger volumes of interest, expanding the capabilities of material modeling significantly.
The Impact on Material Science and Engineering
The ability to accurately predict material properties has profound implications for material science and engineering. By understanding the mechanical behavior of materials at a micro-scale, engineers can design materials with optimized properties for specific applications. This approach not only enhances the performance of materials but also contributes to the development of more sustainable and efficient manufacturing processes.
Future Directions
The successful integration of XSTT and FEM paves the way for further advancements in material analysis and modeling. Future research may focus on refining the imaging and modeling techniques to accommodate a wider range of materials and applications. Additionally, the development of more sophisticated algorithms for data processing and analysis could further enhance the accuracy and efficiency of material property predictions.
The integration of X-ray Scattering Tensor Tomography with Finite Element Modeling marks a significant milestone in the advancement of material property predictions. This innovative approach offers a more detailed and accurate analysis of materials, enabling the design and development of materials with optimized mechanical properties. As this methodology continues to evolve, it holds the promise of revolutionizing the field of materials science and engineering, leading to new discoveries and applications in material design.
References
We'd like to extend our heartfelt thanks to Robert M. Auenhammer, Jisoo Kim, Carolyn Oddy, Lars P. Mikkelsen, Federica Marone, Marco Stampanoni, and Leif E. Asp for their invaluable contributions to the valuable research on integrating X-ray Scattering Tensor Tomography with Finite Element Modeling. Their pioneering work has significantly advanced our understanding and prediction of material properties, marking a notable achievement in the field of material science and engineering.
What's Next!
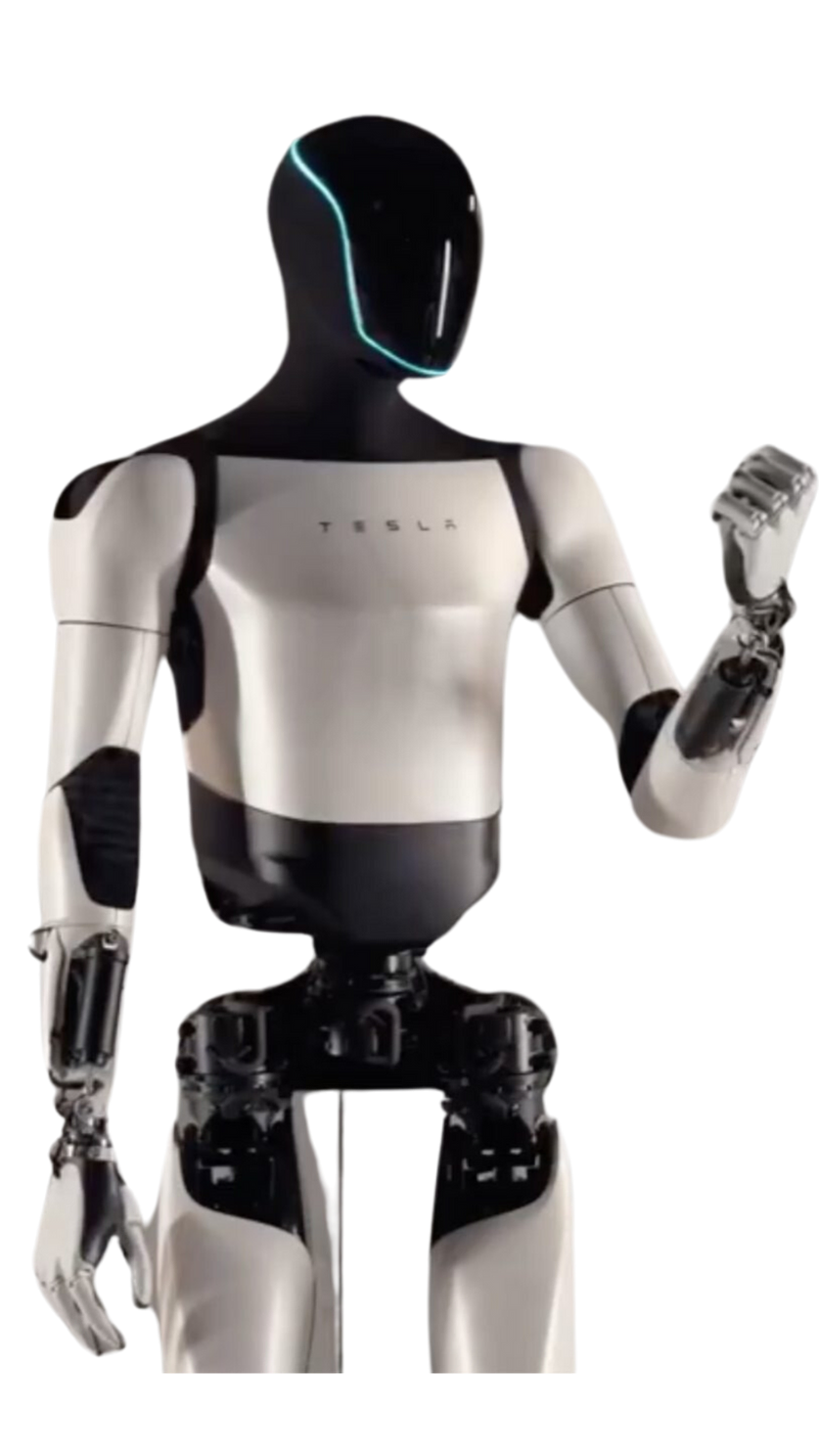
Discover the future of composite manufacturing with Addcomposites! Here's how you can get involved:
Stay Informed: Subscribe to our newsletter to receive the latest updates, news, and developments in AFP systems and services. Knowledge is power, and by staying informed, you'll always have the upper hand. Subscribe Now
Experience Our Technology: Try our cutting-edge simulation software for a firsthand experience of the versatility and capability of our AFP systems. You'll see how our technology can transform your production line. Try Simulation
Join the Collaboration: Engage with us and other technical centers across various industries. By joining this collaborative platform, you'll get to share ideas, innovate, and influence the future of AFP. Join Collaboration
Get Hands-On: Avail our educational rentals for university projects or semester-long programs. Experience how our AFP systems bring about a revolution in composite manufacturing and leverage this opportunity for academic and research pursuits. Request for Educational Rental
Take the Next Step: Request a quotation for our AFP systems. Whether you're interested in the AFP-XS, AFP-X, or SCF3D, we are committed to offering cost-effective solutions tailored to your needs. Take the plunge and prepare your production line for the next generation of composite manufacturing. Request Quotation
At Addcomposites, we are dedicated to revolutionizing composite manufacturing. Our AFP systems and comprehensive support services are waiting for you to harness. So, don't wait – get started on your journey to the future of manufacturing today!
Comments