Real-Time Monitoring of Composite Bonded Joints
- Pravin Luthada
- Jun 5, 2024
- 9 min read
Updated: Aug 12, 2024
TLDR
Understanding Fracture Mechanisms in Composite Bonded Joints
Understanding the fracture mechanisms in composite bonded joints is crucial for improving their performance and durability in various applications, particularly in aerospace and automotive industries. Here is a detailed overview based on the provided sources:
Fracture Mechanisms in Composite Bonded Joints
1. Types of Fracture Mechanisms
Fracture mechanisms in composite bonded joints can be broadly categorized into cohesive and interlaminar fractures. Cohesive fractures occur within the adhesive layer, while interlaminar fractures happen within the composite substrates themselves.
2. Influence of Adhesive and Adherend Properties
The properties of both the adhesive and the adherends significantly influence the fracture behavior. For instance, the fracture toughness and shear behavior of composite bonded joints can be enhanced by using novel aerospace adhesives, which improve the joint's resistance to crack initiation and propagation. Additionally, the nature of the adherends, such as their material composition and fiber orientation, also plays a critical role in determining the joint's toughness.
3. Mode I and Mode II Fracture Toughness
Fracture toughness is often evaluated under different loading modes:
Mode I (Opening Mode): This mode involves the opening of the joint perpendicular to the adhesive layer. Studies have shown that the fracture toughness under Mode I can be significantly affected by the loading rate and environmental conditions.
Mode II (Shear Mode): This mode involves in-plane shear loading. The fracture toughness under Mode II is influenced by factors such as adhesive thickness and the presence of flaws at the bondline.
4. Mixed-Mode Loading
In real-world applications, joints often experience mixed-mode loading, combining both Mode I and Mode II. Research has developed various testing methodologies to evaluate the mixed-mode fracture toughness, such as the use of double cantilever beam (DCB) specimens and other specialized apparatus.
5. Environmental and Loading Conditions
Environmental factors, such as temperature and humidity, can significantly impact the fracture behavior of composite bonded joints. For example, moisture content in the adhesive can reduce the static strength of the joints. Additionally, the loading rate can affect the fracture toughness, with higher rates generally leading to lower toughness values.
6. Damage Mechanisms Under Fatigue Loading
Under fatigue loading, composite bonded joints exhibit various damage mechanisms, including crack initiation and propagation within the adhesive layer and delamination within the composite substrates. These mechanisms are influenced by the cyclic loading conditions and the properties of the adhesive and adherends.
7. Acoustic Emission Monitoring
Acoustic emission (AE) monitoring is a valuable technique for identifying fracture mechanisms in composite bonded joints. AE can detect the onset of damage and monitor crack growth in real-time, providing insights into the fracture processes occurring within the joint.
8. Numerical Modeling and Experimental Validation
Numerical modeling, using techniques such as finite element analysis (FEA), is often employed to predict the fracture behavior of composite bonded joints. These models are validated through experimental tests, which help in understanding the complex interactions between the adhesive and adherends under different loading conditions
Understanding the fracture mechanisms in composite bonded joints is essential for advancing their application in high-stress, safety-critical industries. This knowledge can lead to safer, more reliable, and more efficient designs, ultimately enhancing the performance and utility of composite materials in various applications.
Challenges in Identifying Non-Visible Damage Mechanisms
Identifying non-visible damage mechanisms in composite bonded joints presents significant challenges, critical to ensuring the reliability and safety of these structures. Composite materials, with their complex multi-layered architecture, are prone to a variety of fracture mechanisms that can initiate and propagate under different loading conditions. These mechanisms include matrix micro-cracking, fibre/matrix debonding, fibre pull-out, and fibre breakage, many of which occur below the surface and are not detectable through simple visual inspection.
One of the main challenges is the inherent complexity of the composite materials themselves. Composite substrates often consist of multiple layers with varying fibre orientations (e.g., 0, 90, 45, -45, 60, and -60 degrees), each contributing differently to the overall mechanical behavior. The interaction between these layers can lead to intricate fracture patterns that are difficult to predict and identify without advanced monitoring techniques.
Traditional non-destructive testing (NDT) methods, such as ultrasonic testing and digital image correlation, have limitations in detecting these subtle, non-visible damages. These methods primarily focus on surface-level defects or require extensive post-processing and interpretation, which can be time-consuming and may still miss critical early-stage damage mechanisms.
Acoustic emission (AE) structural health monitoring offers a promising solution by providing real-time data on damage progression within the composite structures. However, interpreting AE data is not straightforward. The AE signals, which are essentially ultrasonic waves generated by the rapid release of energy from localized sources within the material, can be influenced by various factors such as the material's geometry, loading conditions, and environmental noise. Differentiating between the signals from actual damage mechanisms and those from extraneous sources requires sophisticated signal processing and analysis techniques.
Additionally, the use of unsupervised artificial neural networks (ANN) combined with continuous wavelet transforms (CWT) has shown potential in clustering AE signals and assigning physical meaning to them. However, this approach is still under development and requires further validation. The challenge lies in accurately correlating AE signal features (like amplitude, duration, and frequency) with specific damage mechanisms, which demands comprehensive experimental data and advanced analytical models.
Moreover, the complexity increases when different toughening mechanisms are simultaneously triggered. For instance, the highest peak frequency in AE signals does not always represent the most critical damage mechanism. Multiple peak frequencies can be associated with various fracture mechanisms occurring concurrently, such as fibre pull-out involving both matrix cracking and fibre breakage.
Acoustic Emission Structural Health Monitoring Techniques
Acoustic emission (AE) structural health monitoring (SHM) techniques are at the forefront of non-destructive evaluation methods for composite bonded joints. These techniques offer a real-time insight into the damage mechanisms that occur within the material, providing critical data that can help enhance the safety, reliability, and performance of composite structures. Here’s an overview of the approach and its implementation:
Principles of Acoustic Emission
Acoustic emission monitoring involves capturing the ultrasonic waves generated by the rapid release of energy from localized sources within a material. These emissions occur due to micro-fractures, matrix cracking, fibre breakage, fibre/matrix debonding, and other damage mechanisms. The AE method is particularly suited for detecting and characterizing these events because it is sensitive to the subtle changes that occur within the composite material's structure.
Methodology
Sensor Placement and Configuration:
A piezoelectric transducer sensor is strategically placed on the specimen to capture the AE signals.
The sensor setup includes a pre-amplifier to enhance the signal quality, and the data is fed into an acquisition unit for analysis.
Data Acquisition:
During testing, the load and displacement values are synchronized with AE data acquisition to correlate mechanical loading conditions with AE activity.
The AE signals are recorded, capturing key features such as amplitude, energy, counts, duration, rise-time, and root mean square (RMS) values.
Signal Processing and Analysis:
Fast Fourier Transform (FFT) is applied to the recorded waveforms to analyze the frequency domain characteristics of the AE signals.
Principal Component Analysis (PCA) is used to reduce the dimensionality of the large datasets and highlight the most relevant features for clustering.
Clustering and Classification:
An unsupervised artificial neural network (ANN), specifically a Self-Organizing Map (SOM), is employed to cluster the AE signals based on their features.
The k-means algorithm helps define the optimal number of clusters, which are then correlated with specific damage mechanisms.
Continuous Wavelet Transform (CWT):
The Morlet wavelet is used to perform a time-frequency analysis of the AE signals, providing deeper insight into the transient nature of the damage events.
This step helps distinguish between simultaneous damage mechanisms and identify their unique signatures within the AE data.
Implementation and Findings
The implementation of AE SHM techniques in composite bonded joints has revealed several key findings:
Damage Identification: AE can successfully identify and differentiate between various fracture mechanisms, including matrix cracking, fibre pull-out, and fibre/matrix debonding.
Real-Time Monitoring: AE enables continuous monitoring during mechanical testing, allowing for the detection of early-stage damage and the observation of its progression.
Complex Damage Patterns: The method is effective in capturing the complex interactions between different damage mechanisms, which traditional NDT methods might miss.
Challenges and Future Directions
Despite its advantages, AE monitoring faces challenges such as high background noise and the need for sophisticated signal processing techniques to accurately interpret the data. Future research is focused on refining these techniques and integrating them into comprehensive SHM systems for real-world applications.
AE structural health monitoring offers a powerful tool for understanding and improving the fracture behavior of composite bonded joints. By providing detailed insights into the internal damage mechanisms, AE techniques help enhance the design, reliability, and safety of these critical components.
Enhancing Fracture Toughness and Reliability in Composite Joints
Improving the fracture toughness and reliability of composite adhesively bonded joints is a critical goal in the aerospace and transportation industries. Composite materials, known for their lightweight and high-strength properties, require advanced techniques to ensure their durability and performance in high-stress applications. This section discusses the approaches and solutions developed to enhance the fracture toughness and reliability of these joints.
Tailoring Composite Layup Sequences
One effective method to enhance the fracture toughness of composite joints is by tailoring the composite layup sequences. By adjusting the orientation and sequence of the fibre layers within the composite substrate, different toughening mechanisms can be triggered. These mechanisms, both intrinsic and extrinsic, influence crack propagation paths and can delay crack advancement, thus improving the joint's overall toughness.
Example Layup Sequences:
[0]8: Unidirectional fibres aligned in one direction.
[0/902/0]S: A symmetric layup with 0° and 90° fibre orientations.
[90/45/-45/0]S: A complex layup including 45° and -45° fibre orientations to enhance multi-directional toughness.
Acoustic Emission Monitoring
Acoustic Emission (AE) monitoring is a powerful tool for identifying damage mechanisms in real-time. This technique captures the ultrasonic waves generated by damage events within the material, providing insights into the initiation and progression of fractures.
Key AE Monitoring Techniques:
Principal Component Analysis (PCA): Reduces the dimensionality of AE data, highlighting the most significant features for clustering.
Continuous Wavelet Transform (CWT): Analyzes the time-frequency domain of AE signals, distinguishing between different damage mechanisms.
Use of Toughened Epoxy Adhesives
The choice of adhesive significantly impacts the joint's performance. Toughened epoxy adhesives, such as AF163-2K, are designed to improve the fracture toughness of bonded joints. These adhesives incorporate toughening agents that enhance their ability to absorb energy during fracture, reducing the likelihood of sudden catastrophic failures.
Example Adhesive Properties:
GIC (Critical Strain Energy Release Rate): 2416 J/m², indicating high toughness.
Non-Destructive Testing (NDT) Techniques
NDT techniques are essential for assessing the integrity of composite joints without causing damage. Combining AE monitoring with traditional NDT methods, such as X-ray micro-CT scanning, provides a comprehensive view of the joint's condition.
X-ray Micro-CT Scanning: Offers high-resolution images of the internal structure, revealing hidden defects and damage mechanisms.
Integrating Machine Learning
Machine learning algorithms, particularly unsupervised learning models like Self-Organizing Maps (SOMs), are increasingly used to analyze AE data. These algorithms can cluster AE signals into groups corresponding to different damage mechanisms, improving the accuracy of damage detection and assessment.
Future Directions
Continued research and development in these areas aim to further enhance the fracture toughness and reliability of composite joints. Key focus areas include:
Real-Time Monitoring Systems: Developing integrated SHM systems that provide continuous, real-time monitoring of composite structures in-service.
Advanced Material Designs: Innovating new composite materials and adhesives with superior toughness and reliability.
Improved Analytical Models: Refining the algorithms and models used to interpret AE data, increasing their accuracy and applicability.
References
I would like to thank the authors R.A.A. Lima, R. Tao, A. Bernasconi, M. Carboni, and S. Teixeira de Freitas for their invaluable contributions in the study detailed in the PDF "Acoustic Emission Approach for Identifying Fracture Mechanisms in Composite Bonded Joints: A Study on Varying Substrate’s Stacking Sequence." Their meticulous research and insightful findings have significantly advanced our understanding of fracture mechanisms in composite bonded joints. The innovative use of acoustic emission techniques combined with sophisticated data analysis methods has paved the way for more reliable and safer applications of composite materials in critical industries.
What's Next!
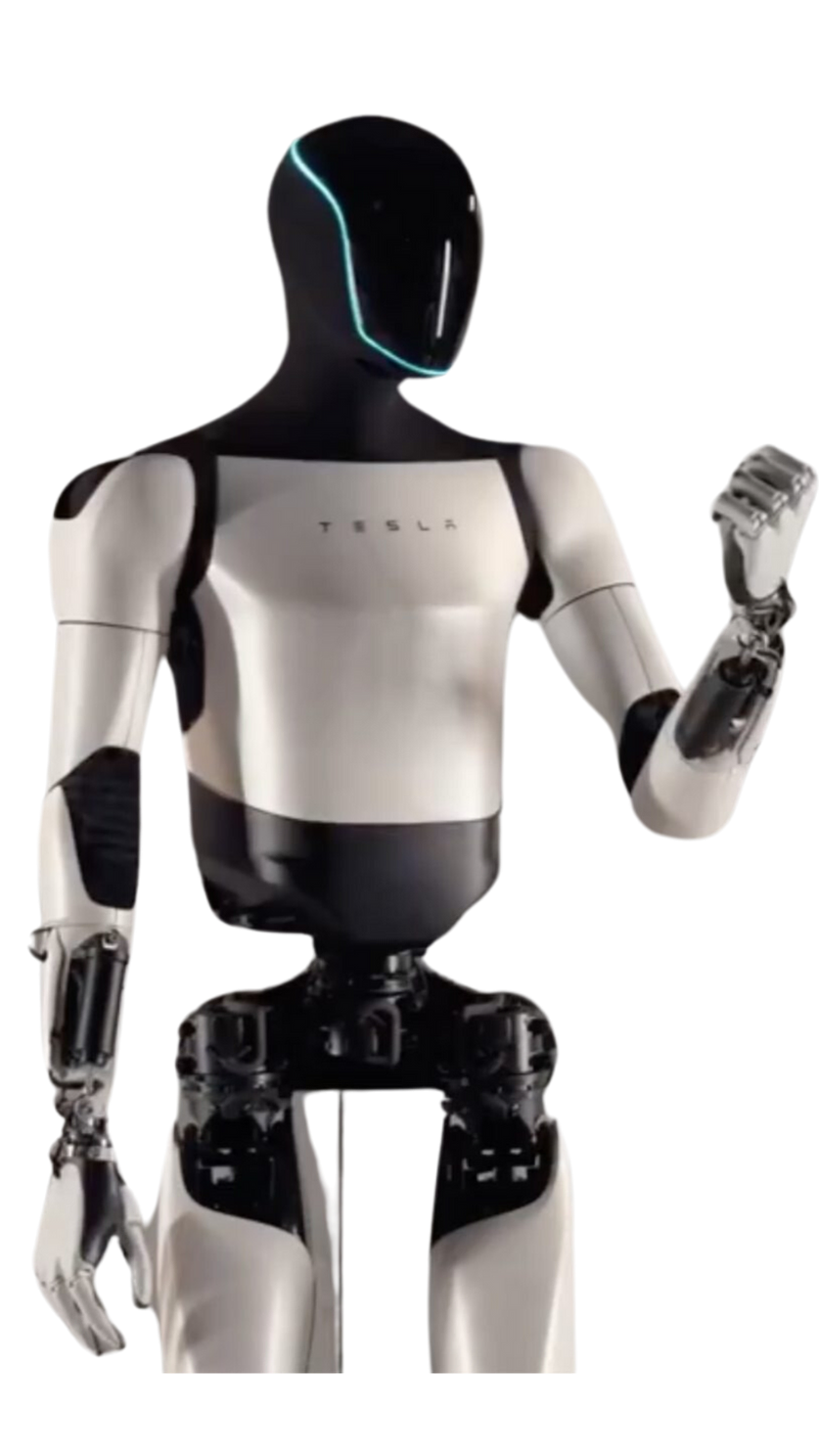
Discover the future of composite manufacturing with Addcomposites! Here's how you can get involved:
Stay Informed: Subscribe to our newsletter to receive the latest updates, news, and developments in AFP systems and services. Knowledge is power, and by staying informed, you'll always have the upper hand. Subscribe Now
Experience Our Technology: Try our cutting-edge simulation software for a firsthand experience of the versatility and capability of our AFP systems. You'll see how our technology can transform your production line. Try Simulation
Join the Collaboration: Engage with us and other technical centers across various industries. By joining this collaborative platform, you'll get to share ideas, innovate, and influence the future of AFP. Join Collaboration
Get Hands-On: Avail our educational rentals for university projects or semester-long programs. Experience how our AFP systems bring about a revolution in composite manufacturing and leverage this opportunity for academic and research pursuits. Request for Educational Rental
Take the Next Step: Request a quotation for our AFP systems. Whether you're interested in the AFP-XS, AFP-X, or SCF3D, we are committed to offering cost-effective solutions tailored to your needs. Take the plunge and prepare your production line for the next generation of composite manufacturing. Request Quotation
At Addcomposites, we are dedicated to revolutionizing composite manufacturing. Our AFP systems and comprehensive support services are waiting for you to harness. So, don't wait – get started on your journey to the future of manufacturing today!
Kommentare